A team of College of Optics and Photonics (CREOL) researchers believe photonic technology could be the key to getting deeper, clearer views into the universe than ever before — and their work is creating new opportunities for those who want to reach for the stars.
Led by Professor Stephen Eikenberry, the team has secured nearly $3.5 million in funding from a MURI (Multidisciplinary University Research Initiatives) grant through the Air Force Office of Scientific Research. Researchers will investigate new ways to view the cosmos —but the applications don’t only apply to the deepest reaches of outer space.
“We’re collaborating on the photonic technologies that we could apply to exploring the solar system, looking for life on Europa, and doing some of the work we need to do to colonize the moon and Mars,” Eikenberry says.
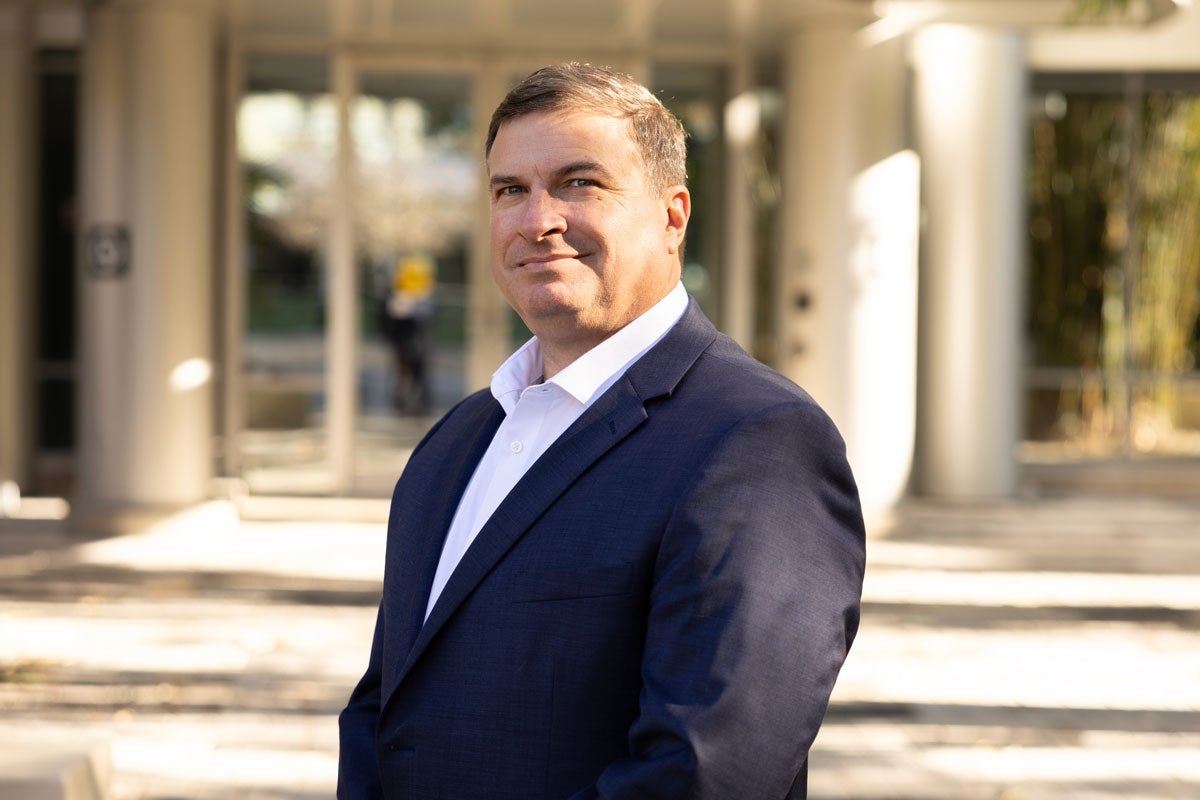
And the list goes on, from accurately identifying and characterizing space debris, to improving communication between satellites, to tracking stars and planets that orbit black holes.
“We could potentially image forming planets around baby stars in a way that hasn’t been done before,” Eikenberry says.
The team’s research focuses on two key technologies: photonic lanterns and heterodyne spectroscopy. By capturing, measuring and combining light waves in specific ways, researchers can synthesize images at scales and resolutions never seen before, further unlocking the mysteries of our reality.
Nearly a third of CREOL’s faculty members are involved, including professors Rodrigo Amezcua-Correa, Miguel Bandres, Peter Delfyett, Darren Hudson and Axel Schülzgen. As of Spring 2025, the CREOL astrophotonics team includes more than 20 undergraduate students and 15 doctoral students. One of them is Tara Crowe, who says she decided to pursue her doctorate at UCF because of the program.
“You either do optics, or you do astronomy,” Crowe says. “And I really wanted to do both. Surprisingly, there are very few programs that do both.”
The research funding will also make an impact beyond CREOL, providing opportunities for physics students to participate in the projects.
“CREOL has a very strong history of innovating brand-new technologies,” Eikenberry says, “And we’re getting some traction. We’re getting some real motion there.”
A New Type of Telescope
Imagine a telescope with an aperture the size of the entire Earth. What would we be able to see?
But before looking up, Eikenberry says look down — at your car stereo.
“Your radio in your car — you tune it. It’s got an oscillator,” Eikenberry says. “You turn your dial, and that’s adjusting your frequency. Meanwhile, you’ve got an antenna that’s receiving all the stuff.”
When the frequency being received by the antenna matches the frequency on your dial, you hear a clear sound.
This roughly describes how the Event Horizon Telescope works to observe the cosmos. It’s a network of radio telescopes around the globe that combine their individual signals to produce a single image. This is how the first direct image of a black hole was produced in 2019.
Radio waves are a form of electromagnetic radiation, or simply, light. The CREOL team’s goal is to apply the same concept the Event Horizon Telescope uses — but for infrared light.
“A lot more interesting things give off infrared or visible light, than give off radio waves,” Eikenberry says.
For example, the James Webb Space Telescope has produced world-famous infrared images, including Webb’s First Deep Field, the deepest infrared look into the universe to date.
Eikenberry says creating a global array of infrared telescopes will produce images with a resolution 10,000 times greater than radio telescopes can. However, combining and processing the telescopes’ data will require some photonic innovation.
CREOL is working with five other institutions as part of a Multidisciplinary University Research Initiative (MURI), including The University of Texas at Austin, University of Colorado Boulder, Yale University, University of Southern California and University of Michigan. The Air Force Office of Scientific Research is overseeing the award sponsored by the U.S. Department of Defense.
Eikenberry and Delfyett have been allocated $2 million to investigate the concept.
Delfyett says the key to “tuning in” the telescopes lies in laser frequency combs.
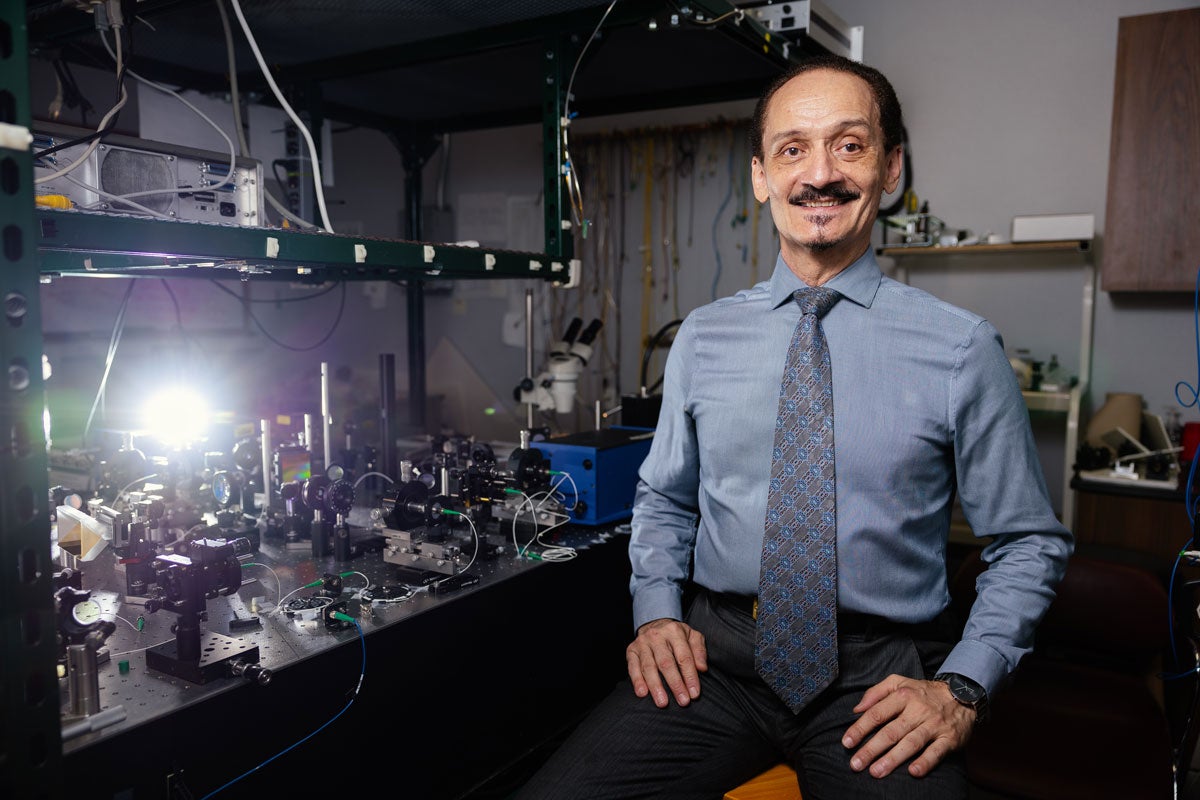
“A laser frequency comb is a light source that produces many different colors,” Delfyett says. “And the different colors add together to make very short bursts of light, which can be utilized for length or time metrology, meaning that we’re able to measure the distance between these two telescopes.”
And that precision is paramount.
“If we want these telescopes on the opposite side of the Earth to be able to look at some object, take the information and add it together to have improved resolution, we must know that distance to the accuracy of the wavelength of light.”
The result: an infrared or near-infrared telescope with a virtual aperture the size of our planet, or bigger.
“This will give us an unprecedented ability to explore and measure the cosmos,” Delfyett says.
Photonic Lanterns Light the Way
Delfyett says the same technology can be used to look back down on the Earth. Using a constellation of small satellites, the images that could be captured would have the same signature, ultra-high resolution.
This technology has broad applications, including defense and environmental science. Delfyett says it could provide new high-resolution looks at forests, jungles, deserts and even three-dimensional cloud structures.
On the ground, Eikenberry says the telescope array could provide the same close-up view of objects in orbit, including a precise observation not just of the outside — but the inside of the International Space Station, which is in low-Earth orbit.
“With this technology, we would look through the window and read the 10-point font of the text message a person just got from his wife back home,” he says.
Eikenberry says this could prove useful in identifying cracks and other defects on expensive equipment high above the Earth’s surface, which are major concerns for the safety of people and equipment in orbit.
“A piece of space debris the size of a big marble can knock out your billion-dollar satellite,” Eikenberry says. “And right now, with the classical diffraction limit, even with adaptive optics to remove the blurring of the atmosphere, you still only get images that are limited by the diffraction of the telescope. You see a blurry thing about the size of a softball, you don’t know, is that a collection of little rocks? Is it a ball of dust? A ball of dust you don’t really care too much about, but a bunch of rocks matters.”
This is where photonic lanterns come in. While the technology has existed for a while, Eikenberry’s team is now researching how to use them as quantum-inspired imagers to attain the high resolution necessary to characterize space debris. Considering light as a wave, photonic lanterns can measure both the brightness and phase of the light, which Eikenberry says is necessary to sharpen the picture.
Looking further out into the depths of space, while we can detect many distant objects (using radio telescopes and other methods), we can’t necessarily produce a visual image of them. Advances in photonic lanterns could change that.
Eikenberry points to the formation of new planets around other stars: “Right now, we kind of know they’re there by looking at their spectral signatures, but we could be able to actually take a picture and resolve it — and figuring that out would be a huge leap forward.”
Using this light-based technology could also lead to more answers about the darkness in the universe. Black holes, Eikenberry says, can accumulate material from neighboring stars, and shoot it out in a stream that moves at 99% of the speed of light — but why that happens remains unclear.
“It’s a big mystery that’s been around since the 1960s,” he says, “Why does it shoot out little, narrow streams of material out of the deepest potential wells in the universe?”
Capturing visual and infrared images could lead to new revelations in astronomy, astrophysics and even astrobiology, the researcher says.
The Future of Space Photonics
Eikenberry says the spirit of collaboration at CREOL is what makes the advances in this emerging field possible.
“We’re sort of tying it all together now and making this big push into space photonics and astrophotonics, in particular,” Eikenberry says.
The expanding research areas support UCF President Alexander N. Cartwright’s mission to strengthen the university’s position as a leader in space research. This is implemented through the Space and Planetary Instrumentation, Commercialization, and Education (SPICE) initiative, managed by the Florida Space Institute providing support to multiple colleges and departments, including CREOL for the advancement of space photonics. The SPICE project aims to attract even more funding by empowering researchers with the resources and facilities they need to conduct advanced experiments for space research and develop emerging space technologies.
CREOL also has plans to hire a space photonics instructor, and the program’s growth will allow more students to explore their passion for the space industry.
Crowe says her work on the team as a CREOL student means more than just making progress toward her doctorate.
“It makes you feel like you’re doing something that’s going to take you somewhere,” Crowe says.
About the Researchers
Eikenberry earned his doctoral degree in astronomy at Harvard University in 1997. He joined CREOL in 2021 after serving as a professor of astronomy at the University of Florida and before that, Cornell University. Eikenberry’s research group at CREOL focuses on studying black holes, neutron stars, dark energy and extrasolar planets. He also specializes in building astronomical instruments to observe them.
Delfyett has authored more than 200 scientific publications, holds 45 U.S. patents, and was recently inducted into the Florida Inventors Hall of Fame. He is UCF’s first sitting faculty member to be inducted into the National Academy of Engineering. He serves as the Director of the Townes Laser Institute and his contributions have been extensive, spanning the underlying physics, device development and application of semiconductor-based mode-locked laser diodes.